All published articles of this journal are available on ScienceDirect.
The Higher Frequency of Blood Group B in a Brazilian Population with HIV Infection
Abstract
Objective:
To analyze the frequency of and odds for and against HIV infection based on ABO blood type in a large sample of blood donors.
Background:
Coevolution between pathogens and hosts may explain the ABO system of polymorphisms. HIV-infected cells add ABO(H) blood group antigens to the viral envelope. Naturally occurring antibodies against ABO(H) antigens that are present in normal human sera are able to neutralize ABO-expressing HIV in vitro. Blood donors are ideal for studying blood groups and HIV infection in vivo because all donors are routinely typed and tested.
Methods:
All blood donors who donated blood between 1994 and 2010 were tested for HIV (ELISA antibody tests and Western blot test or immunofluorescence testing) and were ABO typed (direct and reverse grouping tests). HIV infection based on the ABO blood group was analyzed using the chi-square test and game theory.
Results:
The total number of examined blood donors during this period was 271,410, of whom 389 were infected with HIV. B-group donors were more infected than non-B donors (p= 0.006).
Conclusions:
A more restricted antigen recognition capacity of anti-Galα1-3Gal in blood groups AB and B and a weaker antigen-binding capacity of anti-A antibodies may contribute to a higher frequency of HIV infection in blood group B.
INTRODUCTION
Polymorphic blood group antigens expressed on the surfaces of epithelia, red cells, platelets and granulocytes which are exploited as attachment receptors by HIV, are associated with resistance against infection [1-4]. Neutralization and first-line immune recognition of pathogens by natural or spontaneous antibodies are also important in resistance against infection [5]. Most high-titer antibodies in humans can recognize glycan antigens of the ABO histo-blood group and galactosylα1-3 galactose (Galα1-3Gal), which represent 1% of the total IgG [6]. Antibodies against Galα1-3Gal are found in all humans, great apes and monkeys of the Old World, while antibodies against N-acetylgalactosamine (blood group A) and D-galactose (blood group B) are expressed in a polymorphic manner. The production of these highly reactive antibodies is induced by glycans that are synthesized in epithelia and expressed on the cell surface by related glycosyltransferases localized in the Golgi apparatus [7, 8]. The lack of Galα1-3Gal and ABO antigens induces the biosynthesis of potent complement-activating and neutralizing antibodies early in life [7, 8]. Anti-Galα1-3Gal antibodies found in individuals of blood groups A and O have a broader cross-reacting antigen recognition capacity, identifying and binding to B antigens, whereas AB- and B-group individuals produce anti-Galα1-3Gal antibodies with a more restricted antigen recognition capacity, binding only to Galα1-3Gal [9]. Coevolution between hosts and pathogens likely contributes to the polymorphism of ABO glycans [10, 11].
HIV envelope glycosylation patterns are defined during passage through the Golgi apparatus and are responsible for the immune evasion of the virus [12, 13]. ABO glycosyltransferases that are present in HIV-infected cells add ABO blood-group glycans to glycoprotein 120 (gp120) [14]. Anti-ABO blood-group antibodies that are present in normal human sera are able to neutralize in vitro ABO-expressing RNA viruses, such as HIV and measles virus, in a complement-dependent manner [14-16]. The successful viral strategy of avoiding innate immune recognition by hiding the viral envelope within host glycans would theoretically fail when invading a new host without this glycan profile and who therefore would possess antibodies against the virus. One explanation for both the selection in favor of rare ABO alleles and the frequency distribution of ABO phenotypes may be found in the modeled evolution of ABH polymorphism by two different pathogenic strategies that act simultaneously: (i) bacterial pathogens exploiting cell-surface glycans as receptors; and (ii) intracellular viruses exploiting the host glycan profile of the ABO blood group to evade the action of anti-ABH antibodies [17]. Immune evasion and recognition are strategies employed by pathogens and hosts during coevolution. Evolutionary game theory is therefore an appropriate theory for analyzing the outcomes of these strategies [18].
Our hypothesis is that the frequency of HIV infection should be different for different ABO phenotypes. Considering the lack of studies addressing this issue, the aim of the present study was to analyze the frequency of HIV infection and the odds of being infected in a population of blood donors according to donors’ ABO blood group antigen and antibody profile. Blood donors are ideal for this purpose, as all donors are routinely subjected to ABO blood group antigen and antibody typing and laboratory tests to detect HIV infection.
MATERIALS AND METHODS
We analyzed all blood donors who donated between January 1994 and October 2010 at the Blood Bank of the Hospital de Clínicas de Porto Alegre, a 700-bed teaching hospital located in southern Brazil. The hospital serves patients and blood donors from the state of Rio Grande do Sul, which has a current population of 10,693,929 (Instituto Brasileiro de Geografia e Estatística (IBGE), 2010) [19]. The study was approved by the Institutional Review Board and was conducted in accordance with current Brazilian legislation.
All blood donors underwent clinical evaluation, which excluded individuals at risk for sexually transmitted diseases, drug use, anemia, infection, fever, or chronic diseases. Blood donors who were positive in two different ELISA antibody tests and one Western blot test, or those who were positive in one immunofluorescence test for HIV-1 or -2, were considered infected (Abbott Murex HIV 1.2.0, Dartford, USA). The ABO blood group for each donor was determined by direct slide and tube agglutination with donor red blood cells and anti-A and -B antibodies and confirmed by tests using anti-A and -B reagents in the blood donor serum samples (DMS Laboratories, Flemington, NJ, USA).
Frequencies of blood groups were calculated for total, HIV-negative and -positive donor populations. Blood donors were compared using the Kruskal-Wallis test for age and the chi-square test for categorical variables. Odds ratios (OR) for HIV infection and the respective 95% confidence interval (CI) for blood groups were also estimated. Statistical analyses were performed using the Statistical Package for the Social Sciences (SPSS) version 15.0. Odds for and against HIV infection based on ABO blood group were used as an outcome measure (payoff) to construct a game matrix to analyze the evolutionary dynamics of immune evasion and recognition strategies. Odds ratios were obtained by calculating the probability (p) of infection based on the ABO blood group, dividing the number of infected donors by the total number of donors. The probability of not being infected was calculated as 1-p. Odds for infection were calculated as p/(1-p) and against infection as (1-p)/p.
RESULTS
The total number of blood donors during this period was 271,410, of whom 271,021 were not infected with HIV and 389 were infected with HIV (males: 279; females: 110; mean age: 32.6 years; 95% CI: 31.7-33.5). There were no differences in age or gender between ABO blood groups. The highest frequency of infected donors was observed in blood group B (0.206%), followed by A (0.138%), O (0.137%) and AB (0.113%). The odds of finding an HIV-infected blood donor was higher in blood group B (0.00206), thus making the odds of finding an HIV-negative donor lower in this blood group (485.2) (Table 1).
Number and Frequency of Blood Donors and Probability and Odds for and Against HIV Infection According to ABO Blood Group
A | AB | B | O | Total | |
---|---|---|---|---|---|
All Donors | |||||
N | 106,757 | 8,855 | 26,255 | 129,543 | 271,410 |
Frequency | 0.39334 | 0.03263 | 0.09674 | 0.47730 | 1.00000 |
HIV-Negative | |||||
N | 106,610 | 8,845 | 26,201 | 129,365 | 271,021 |
Frequency | 0.39336 | 0.03264 | 0.09668 | 0.47732 | 1.00000 |
HIV-Positive | |||||
N | 147 | 10 | 54 | 178 | 389 |
Frequency | 0.37789 | 0.02571 | 0.13882 | 0.45758 | 1.00000 |
Probability | |||||
Infected | 0.00138 | 0.00113 | 0.00206 | 0.00137 | 0.00143 |
Not infected | 0.99862 | 0.99887 | 0.99794 | 0.99863 | 0.99857 |
Odds | |||||
Infected | 0.00138 | 0.00113 | 0.00206 | 0.00138 | 0.00144 |
Not infected | 725.2 | 884.5 | 485.2 | 726.8 | 696.7 |
The frequency of HIV infection was different between blood groups (chi-square test: p = 0.041). The overall chi-square statistics for a contingency table can always be partitioned into as many components as the table has degrees of freedom [20]. In the present investigation, we compared HIV(+) prevalence between blood groups B and non-B, between AB and non-A, and between O and A (Table 2). There was a higher representation of HIV-infected individuals among B-group donors when compared to non-B donors. (Fisher’s exact test: p = 0.006; OR for HIV+ B individuals compared to non-B: 1.506; 95% CI: 1.130-2.008). As a further precaution, p-values were multiplied by 3 to allow for multiple comparisons (Bonferroni correction), and in this case, ignoring prior knowledge, p was 0.018, which still supports the conclusion that there are more HIV(+) individuals among B-group individuals at a statistically significant level (Table 2).
Association Between HIV Infection and Blood Groups: Partition of the Overall Chi-Square Statistics
Comparison (Blood Groups) | Chi-Square Test | DF | p | Adjusted p-Value (Bonferroni Correction) |
---|---|---|---|---|
Overall (A vs AB vs O vs B) | 8.257 | 3 | 0.041 | |
B vs non-B | 7.895 | 1 | 0.006 | 0.016 |
AB vs non-A | 0.591 | 1 | 0.442 | 1.000 |
O vs A | 0.0004 | 1 | 0.985 | 1.000 |
DF = degree of freedom.
Immune evasion and recognition are important in the coevolution between pathogens and host populations. The simultaneous analysis of odds in favor and against finding an HIV-infected donor based on relevant phenotype estimates was aimed at detecting outcomes and the consequent success of the adopted immune strategies. A game matrix (using the values previously described in Table 1) was constructed to analyze the outcomes for HIV infection (odds of being infected) and donors (odds of not being infected) rooted in the respective donor blood group: B (anti-A), A (anti-B), O (anti-A and anti-B) and AB (no anti-A or anti-B) (Fig. 1).
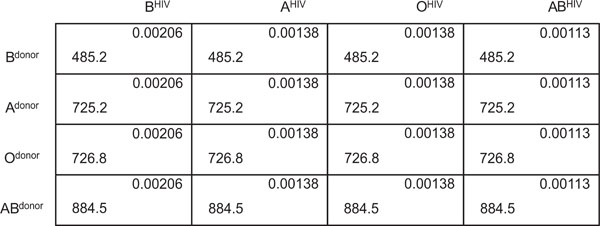
Odds for HIV infection (top right corner of each square) (BHIV > AHIV ≥ OHIV ≥ ABHIV) and odds against HIV infection (lower left corner of each square) (ABdonor ≥ Odonor ≥ Adonor > Bdonor) according to blood groups.
According to game theory, the best HIV infection strategy was to invade B-group hosts, and the best donor strategy for non-infection was to belong to a non-B blood group.
DISCUSSION
The frequency of HIV infection with respect to antigens and antibodies against the polymorphic ABO histo-blood group was analyzed in 271,410 blood donors. The highest proportion of HIV-infected individuals was observed in blood group B, in which only anti-A antibodies were present. The lowest proportion was observed in groups O and A, in which anti-B and cross-reacting anti-Galα1-3Gal antibodies were present. Surprisingly, a small proportion of HIV-infected individuals was observed in blood group AB, in which both anti-A and -B antibodies were absent. This finding may in part be explained by the fact that we studied clinically asymptomatic blood donors, whose diagnosis of HIV infection was made after blood donation. A previous study in a small number of HIV-infected patients have shown that subjects with blood group B and also those who are Rh negative show decreased susceptibility to HIV infection [21]. Other infectious diseases, such as Chagas’ disease and dengue fever, have a more aggressive and symptomatic course in AB-group individuals [22, 23]. Infection with HTLV-1 has also been more frequently observed in blood group AB [24]. A higher proportion of HIV-infected AB-group individuals could therefore develop symptoms earlier in life, which would consequently exclude them from blood donation. A second possibility is that the simultaneous expression of A- and B-glycans in epithelia may reduce HIV receptor recognition or attachment.
B-group donors with only anti-A antibodies had the lowest odds against HIV infection (473.4), which may be explained by the superiority of anti-B activity in hosts with anti-B antibodies [9]. Cross-reacting anti-Galα1-3Gal antibodies present in blood groups A and O show a fourfold higher anti-B activity than specific anti-B antibodies. Anti-Galα1-3Gal antibodies that are present in blood group B do not cross-react with A antigens. Anti-A antibodies bind more weakly to A antigens than anti-B antibodies bind to B antigens because of the greater antigenic heterogeneity of blood group A [25]. This superior anti-B activity may also explain why the ABO*B allele is less frequent than the ABO*A allele in most populations. Antibodies against ABH glycans and the related Galα1-3Gal appear early in life, without previous exposure to pathogens. These antibodies are the most potent activators of the lytic complement system in humans and the primary barrier to allo- and xenotransplantation and transfusion. Host antibodies recognize and neutralize viral pathogens expressing non-self antigens, thus preventing intracellular invasion and dissemination to vital organs [5]. This is an important strategy of the innate immune system, as it is independent of prior exposure to pathogens. The HIV infection strategy of using the glycan profile of the infected host to evade immune recognition and neutralization is so successful that 100% of infected individuals develop a chronic and potentially lethal disease. This strategy, however, is a major obstacle for producing an effective vaccine against HIV [13]. The immune barrier, consisting of pre-existing anti-glycan antibodies is, therefore, important for preventing infections from which the host is unable to recover.
The odds of succeeding in infecting a new host appear to be influenced by the glycan profile expressed on the viral envelope and the repertoire of anti-glycan antibodies of the host. Thus, the antibody asymmetry of a host population may potentially result in transmission asymmetry.
In summary, the more restricted antigen recognition capacity of anti-Galα1-3Gal found in blood groups AB and B, as well as the weaker antigen-binding capacity of anti-A antibodies, may contribute to a higher frequency of HIV infection in B-group donors [25]. The asymmetry of antibodies against ABH glycans and the consequent transmission asymmetry of pathogens expressing ABH glycans may potentially affect the dynamics of viral epidemics based on ABO phenotype frequency in a particular population.
CONFLICT OF INTEREST
The authors confirm that this article content has no conflict of interest.
ACKNOWLEDGEMENTS
Tor Gunnar Hugo Onsten is the main author responsible for the concept and design of the study, discussion and conclusions. Sidia M. Callegari-Jacques organized the presentation of data and critically analyzed the mathematical formulas and statistical results. Luciano Z. Goldani participated in the drafting of the manuscript and contributed substantially to the discussion and conclusions. All authors have read and approved the manuscript as submitted.
No author or related institution has received any financial benefit from research in this study. The authors declare that they have no competing financial interests.